Nunerical Study on Improvement of Airfoil Performance at Low Reynolds Number
JAXA Supercomputer System Annual Report April 2019-March 2020
Report Number: R19EACA43
Subject Category: JSS2 Inter-University Research
- Responsible Representative: Keiichi Kitamura, Associate professor, Yokohama National University
- Contact Information: Suguru Ogawa(ogawa-suguru-ps@ynu.jp)
- Members: Keiichi Kitamura, Suguru Ogawa, Yoshikatsu Furusawa
Abstract
As a new form of vheicle of the Mars explolation, research and development of Mars airplanes are progressing mainly in JAXA in Japan. Since the Martian atmosphere has a low density and Mars airplanes fly in the low Reynolds number region, it is necessary to develop wings that exhibit high flight performance in this low Reynolds number region. In this study, the flow field structure around the wing in the low Reynolds number region is investigated in detail using numerical calculations. This report proposes a method to improve the wing performance and aims to clarify the mechanism.
Reference URL
N/A
Reasons and benefits of using JAXA Supercomputer System
In order to capture fine eddies near the boundary layer in laminar flow calculations, large-scale calculations with a grid exceeding 50 million cells are required, and JSS2 was used to greatly reduce the calculation time. In addition, JSS2 was used to perform a large-scale numerical calculation of the three-dimensional rotor using the flow analysis solver “rFlow3D” for the rotor developed by JAXA.
Achievements of the Year
A moving surface method was introduced as a method to suppress the laminar separation, and its effect was demonstrated with a three-dimensional wing (without considering the wingtip vortex). The moving surface method controls the flow near the surface by moving the wing surface in the direction along the flow. This flow is based on the Couette flow. This method is applied to a part near the wing surface to control the separation by supplying momentum enough to overcome the adverse pressure gradient (see Fig. 1). The calculation conditions are Re = 23000, Mach number 0.2, angles of attack 3, 6, 9 degrees. Here, the airfoil is Ishii wing and the chord length is 1m. The moving surface speed was set to half that of the uniform flow. From the calculation results, remarkable improvements of the lift-drag ratio were achieved at all angles of attack by applying the surface movement method (3 degree 13.7 -> 19.5, 6 degree 13.0 -> 27.6, 9 degree 6.75 -> 25.8). Figure 2 shows the visualization result of the isosurface of the Q value (Q = 0.5) colored by Cp at an angle of attack of 6 degree as a representative case. From Fig. 2 (a), it can be seen that the flow separated at the leading edge of the wing reattached near the middle of the wing and formed a complicated three-dimensional flow field. However, when the moving surface method was applied [Fig. 2 (b)], the leading edge separation was suppressed. It is considered that the negative pressure increased due to the attached flow at the leading edge of the wing, and as a result, the pressure drag was greatly reduced, which led to a significant improvement in the lift-drag ratio. In addition, it was clarified that the application of the moving surface method suppressed the leading edge separation, which otherwise had produced three-dimensionality, resulted in the approximately two-dimensional flow field. Since the surface movement method is less affected by the surrounding environment than other methods, a certain effect can be expected in the Martian environment.
Additionally, we conducted numerical simulations on the aerodynamic interaction between the propeller and the fixed wing in which the airplane with propeller propulsion flying at low Reynolds number cases. The Reynolds number based on the fixed wing chord is Re = 30000, the free stream velocity is 9 m/s, the advance ratio is J = 0.8, and the angles of attack are from -5 to 15 degrees with 5-degree intervals. The airfoil of the fixed wing is Ishii airfoil (the chord length is 50 mm, and the span length is 200 mm), and the propeller is APC Propellers 6x4E which was developed for a model propeller. The propeller is installed forward the leading edge or backward the trailing edge of the fixed wing of the center of the span length by the same distance as the chord length. As a result, in the case that the propeller is located in front of the propeller (Fr. Prop.), the lift coefficient increased linearly against the angle-of-attack (Fig. 3) because the propeller slipstream suppressed the growth of separation over the fixed wing. Thrust coefficient was about 15 – 35% larger than the case of the propeller only (Fig. 4). On the other hand, in the case that the propeller is located behind the propeller (Rr. Prop.), aerodynamic forces acting on the fixed wing were not much different from the case of the fixed wing only, and thrust coefficient was 55 – 220% larger than the case of the propeller only. However, the azimuthal fluctuation of thrust coefficient was very large because the slipstream of the fixed wing interfered with the propeller and therefore the advance ratio fluctuated locally. These results suggest that the design of an airplane flying at low Reynolds numbers must take into account the aerodynamic force fluctuations caused by the propeller/wing interaction.
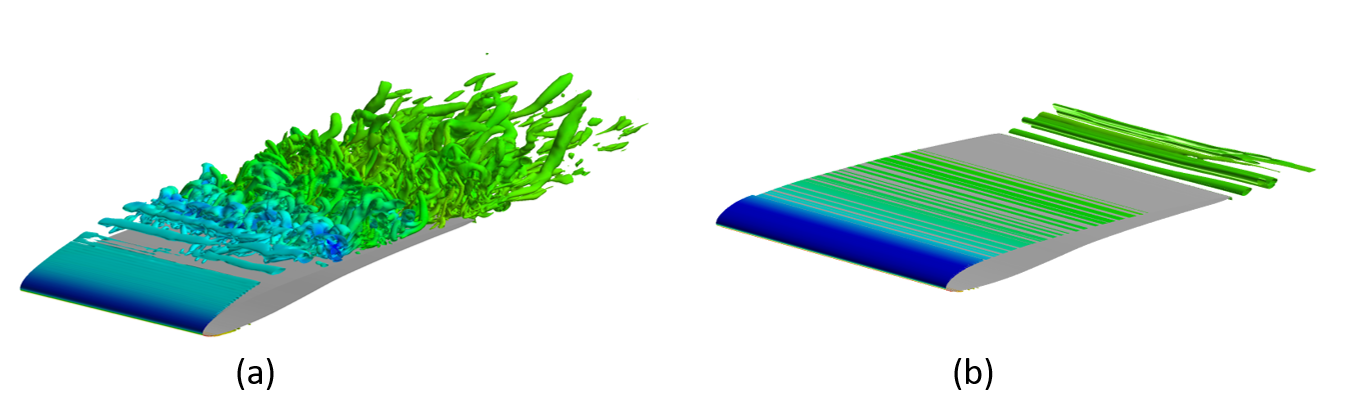
Fig.2: Q iso-surface colored with Cp (-1.5 < Cp < 1.0) (momentary field) (a) without moving surface method (b) With moving surface method
Publications
– Oral Presentations
Suguru Ogawa, Keiichi Kitamura, “Improvement of Low Reynolds Number Aerodynamic Characteristics of Wing by Moving Surface Method,” JSASS-2019-1036, 2019 (in Japanese)
Yoshikatsu Furusawa, Keiichi Kitamura, “Numerical Simulation on Wing/Propeller Interaction by Tractor Propeller,” JSASS-2019-1027, 2019 (in Japanese)
Yoshikatsu Furusawa, Keiichi Kitamura, “Numerical Investigation on Unsteady Aerodynamic Characteristics of Propeller/Wing Interaction at Several Angles-of-Attack,” JSASS-2019-4539, 2019 (in Japanese)
Suguru Ogawa, Keiichi Kitamura, “Improvement of the Aerodynamic Characteristics of Wing by Moving Surface Method at Low Reynolds Number”, 32nd International Symposium on Space Technology and Science (ISTS), 2019
Yoshikatsu Furusawa, Keiichi Kitamura, “Unsteady Numerical Simulation on Angle-of-Attack Effects of Tractor-Propeller/Wing and Pusher-Propeller/Wing Interactions,” AIAA Science and Technology Forum and Exposition 2020, 2020
Usage of JSS2
Computational Information
- Process Parallelization Methods: MPI
- Thread Parallelization Methods: OpenMP
- Number of Processes: 512 – 2048
- Elapsed Time per Case: 140000 Second(s)
Resources Used
Fraction of Usage in Total Resources*1(%): 0.28
Details
Please refer to System Configuration of JSS2 for the system configuration and major specifications of JSS2.
System Name | Amount of Core Time(core x hours) | Fraction of Usage*2(%) |
---|---|---|
SORA-MA | 1,496,626.64 | 0.18 |
SORA-PP | 252,879.23 | 1.64 |
SORA-LM | 1,855.79 | 0.77 |
SORA-TPP | 0.00 | 0.00 |
File System Name | Storage Assigned(GiB) | Fraction of Usage*2(%) |
---|---|---|
/home | 6.68 | 0.01 |
/data | 4,901.89 | 0.08 |
/ltmp | 1,367.19 | 0.12 |
Archiver Name | Storage Used(TiB) | Fraction of Usage*2(%) |
---|---|---|
J-SPACE | 2.37 | 0.06 |
*1: Fraction of Usage in Total Resources: Weighted average of three resource types (Computing, File System, and Archiver).
*2: Fraction of Usage:Percentage of usage relative to each resource used in one year.
JAXA Supercomputer System Annual Report April 2019-March 2020