Study on Future Space Transportation System using Air-breathing Engines
JAXA Supercomputer System Annual Report February 2021-January 2022
Report Number: R21EG3205
Subject Category: Research and Development
- Responsible Representative: Kouichi Okita, Director, Research and Development Directorate, Research Unit IV
- Contact Information: Masatoshi Kodera(kodera.masatoshi@jaxa.jp)
- Members: Masaaki Fukui, Mizuki Fujiwara, Tatsushi Isono, Taku Inoue, Masatoshi Kodera, Toshihiko Munakata, Sadatake Tomioka, Masahiro Takahashi, Hidemi Takahashi
Abstract
Recently, reusable rockets have been being studied to reduce the cost of space transportation systems significantly. However, in order to extend the structural lifetime, it is necessary to operate them with relatively low engine power, leading to a decrease in launch capability. Therefore, air-breathing engines such as scramjets and rocket/scram combined cycle engines are promising to compensate the drawback. By using air in the atmosphere as an oxidizer, it becomes highly efficient, and it can be expected to maintain and improve the launch capability even if it is reused. In this project, we will research and develop key technology for practical application of the engine.
Reference URL
N/A
Reasons and benefits of using JAXA Supercomputer System
The following points are raised as problems of engine design by ground experiments. 1) There are limits to reproducing various airflow conditions from takeoff to hypervelocity range. 2) Measured data is limited and complicated three-dimensional flow structure inside the engine can not be well identified. 3) Since the time and cost are limited, it is not easy to change the engine flow path configuration. Therefore, it is indispensable to utilize 3D CFD as a design tool, and a supercomputer is required for performing numerous CFD works efficiently.
Achievements of the Year
(1) RANS analysis of a scramjet combustor with hydrocarbon fuel
RANS simulations were conducted for a supersonic combustor with a cavity using the CH4/C2H4 mixture for a surrogate of thermally decomposed jet fuel. The CFD results showed good quantitative agreement for wall pressure distributions and qualitative agreement for distributions of local equivalence ratio at the combustor exit in comparison with experiment. Sensitivity analyses showed that especially the estimation of total temperature of the air supplied from the facility was important to accurately predict the experiment. The change of the fuel mixture ratio demonstrated the ram-scram mode transition, suggesting that understanding of the thermal decomposition of fuel is an important factor to control the operation mode.(Fig. 1)
(2) CFD to support scramjet engine tests
In the research of scramjet engines, in addition to CFD of the combustor or engine flows, other flow fields, such as that in the engine test facility, are also studied numerically for the purpose of planning the combustion tests and obtaining the information necessary for better understanding of the combustion test data. One of examples of such applications is the CFD for the Mach 6 nozzle flow of Ramjet Engine Test Facility (RJTF) at JAXA Kakuda Space Center. The RJTF can produce a high-speed flow required for the scramjet engine test at the flow condition corresponding to Mach 8 flight at highest by heating up test gas air. An engine model with a maximum length of 3 m can be tested in the low-pressure chamber exhausted by a steam ejector system. The cross-sectional shape of the RJTF nozzle is rectangular. This is because at the early time of the facility use, a test configuration, in which the nozzle boundary layer was taken into the engine model to emulate a boundary layer generated on the hypersonic vehicle airframe. Furthermore, the nozzle wall at the exit is cut off diagonally to fit the shape of a swept-back inlet leading edge of the engine model. In recent years, there have been increasing opportunities for RJTF to conduct free-jet style engine tests that do not incorporate the nozzle boundary layer into the engine model. Since the influence of the rectangular nozzle exit shape on the uniformity of the nozzle flow was concerned, CFD on the RJTF nozzle flow including the low-pressure chamber and the diffuser duct was conducted. Fig.2 shows the Mach number distribution at each cross-section in the nozzle axis direction, in which only the half region in the spanwise direction is shown. Expansion waves are formed from both the bottom plate edge in the height direction and the lower part of the side wall edge in the spanwise direction, expanding the outer edge of the mainstream outward, and the expanding flow region spread into the inside of the mainstream as it goes downstream. Such information is useful for examining the installation position of the engine model and setting up the inflow profile for the corresponding CFD for the engine test.
(3) Development of FADS system
A new algorithm for a flush air data sensing (FADS) system was developed to estimate flight data and attitude angles for a sharp-nosed hypersonic vehicle. The target condition is Mach 5.0 – 7.0 within the angles of attack of 5 degrees. The FADS system relates air data and pressures from surface-mounted pressure ports on the forebody of the vehicle. The estimation algorithm used by the designed FADS system is validated through numerical simulation, which was based on simpified equations. Results indicated that the designed FADS system can estimate the freestream dynaimc pressure within the uncertainty of 5% in the range of targeted flight conditions.
(4) Validation and evaluation of the Hybrid LES/RANS model
Turbulent boundary layers are an important phenomenon in scramjet engine combustors. Therefore, high grid resolution is required inside the boundary layer to solve it. Therefore, the Hybrid LES/RANS model is effective in reducing computational cost by switching to RANS near the wall and to LES away from the wall. The model was validated by a flat plate boundary layer problem, and the accuracy of the calculations and the effects of initial conditions were evaluated for the analysis of turbulent combustion phenomena in a scramjet combustor.(Fig. 3)
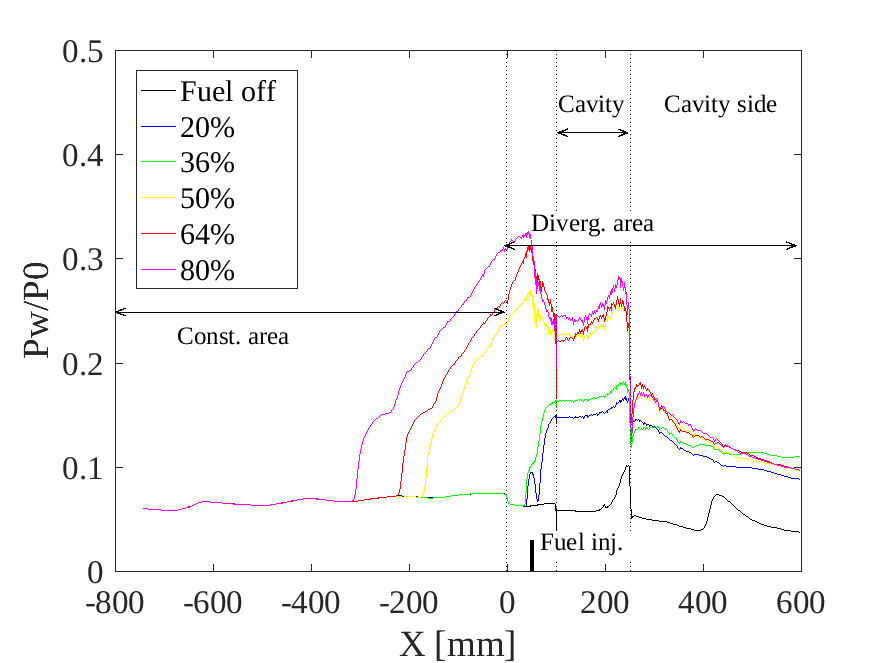
Fig.1: Changes in wall pressure distributions due to ratio of ethylene in the mixed fuel (corresponding to scramjet mode at smaller amount of ethylene, while ramjet mode at larger amount of ethylene)
Fig.3(video): Mach number distribution on a flat plate boundary layer by 3D Hybrid LES/RANS analysis
Publications
– Non peer-reviewed papers
(1) Kodera, M., Tomioka, S., and Takahashi, M., “RANS Simulations of a Supersonic Combustor with Methane/Ethylene Mixed Fuel,” Proceedings of 33rd International Symposium on Space Technology and Science, 2022-a-11, 2022.
(2) Takahashi, H., Taguchi, H., Hirotani, T., Oki, J., and Sato, T., “Design of Flush Airdata Sensing System for Hypersonic Flight Tests with HIMICO Model,” Proceedings of 53rd Fluid Dynamics Conference / 39th Aerospace Numerical Simulation Symposium Online, JSASS-2021-2029-A, 2021.
– Oral Presentations
(1) Kodera, M., Tomioka, S., and Takahashi, M., “RANS Simulations of a Supersonic Combustor with Methane/Ethylene Mixed Fuel,” 33rd International Symposium on Space Technology and Science, 2022.
(2) Takahashi, H., Taguchi, H., Hirotani, T., Oki, J., and Sato, T., “Design of Flush Airdata Sensing System for Hypersonic Flight Tests with HIMICO Model,” 53rd Fluid Dynamics Conference / 39th Aerospace Numerical Simulation Symposium Online, 2021.
Usage of JSS
Computational Information
- Process Parallelization Methods: MPI
- Thread Parallelization Methods: N/A
- Number of Processes: 144 – 4800
- Elapsed Time per Case: 21 Hour(s)
JSS3 Resources Used
Fraction of Usage in Total Resources*1(%): 0.04
Details
Please refer to System Configuration of JSS3 for the system configuration and major specifications of JSS3.
System Name | CPU Resources Used(Core x Hours) | Fraction of Usage*2(%) |
---|---|---|
TOKI-SORA | 228140.73 | 0.01 |
TOKI-ST | 129659.64 | 0.16 |
TOKI-GP | 0.00 | 0.00 |
TOKI-XM | 0.00 | 0.00 |
TOKI-LM | 0.00 | 0.00 |
TOKI-TST | 0.00 | 0.00 |
TOKI-TGP | 0.00 | 0.00 |
TOKI-TLM | 0.00 | 0.00 |
File System Name | Storage Assigned(GiB) | Fraction of Usage*2(%) |
---|---|---|
/home | 390.07 | 0.39 |
/data and /data2 | 30302.57 | 0.32 |
/ssd | 636.29 | 0.16 |
Archiver Name | Storage Used(TiB) | Fraction of Usage*2(%) |
---|---|---|
J-SPACE | 5.37 | 0.04 |
*1: Fraction of Usage in Total Resources: Weighted average of three resource types (Computing, File System, and Archiver).
*2: Fraction of Usage:Percentage of usage relative to each resource used in one year.
ISV Software Licenses Used
ISV Software Licenses Used(Hours) | Fraction of Usage*2(%) | |
---|---|---|
ISV Software Licenses(Total) | 848.42 | 0.59 |
*2: Fraction of Usage:Percentage of usage relative to each resource used in one year.
JAXA Supercomputer System Annual Report February 2021-January 2022